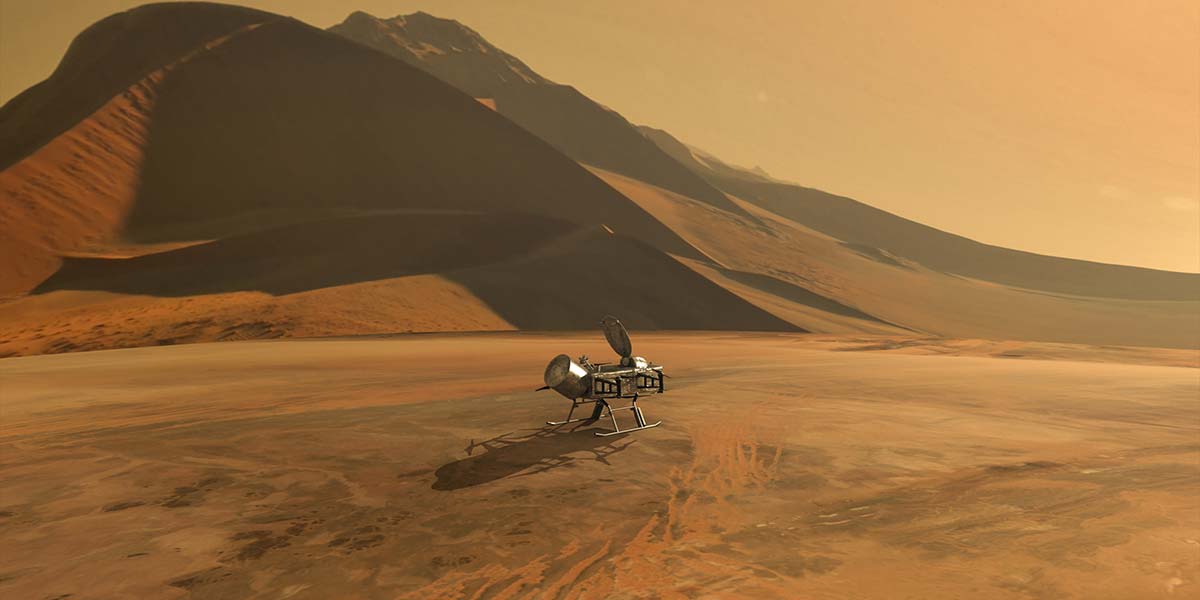
Dragonfly: Game-Changing Exploration of Titan
By Ralph Lorenz View In Digital Edition
Imagine a drone that’s the size of a car, flies itself across a remote landscape using onboard cameras and radar, and has drills and scientific instruments to analyze the materials it finds on that landscape. Then imagine sending such a vehicle a billion miles from home to explore Titan: Saturn’s giant organic-rich moon. Now, stop daydreaming and get to work on the detailed design for the Dragonfly mission!
This is the challenge NASA has given the Johns Hopkins Applied Physics Laboratory (APL): the lab that built the New Horizons spacecraft to go to Pluto (and that will encounter another body, 2014 MU69, in January 2019) and the recently-launched Parker solar probe mission to the Sun.
A couple of times a decade, NASA selects a mission in the New Frontiers Program (https://newfrontiers.larc.nasa.gov) from proposals submitted by the scientific community. Last year, the agency assessed 12 proposals, covering missions to Venus, comets, Saturn and its moons Enceladus and Titan, and the Moon. Of these, NASA selected just two for a detailed mission concept “Phase A” study: Dragonfly, and a comet sample return concept called CAESAR. It has funded each of these Phase A efforts to flesh out mission details during 2018, with the intent to select one of them in the summer 2019 to actually fly; notionally for launch in 2025.
Dragonfly is a concept unlike anything that has ever been proposed before (see http://dragonfly.jhuapl.edu). It’s a relocatable lander that can spend weeks doing science at each site, and then pick itself up to fly up to tens of kilometers in an hour or so to investigate somewhere new. The idea of a small helicopter scout to be based on a lander or rover “mothership” has been suggested in the past and is now set to become a reality with the Mars helicopter to be sent on NASA’s Mars 2020 rover mission. Titan’s environment — with its low gravity and dense atmosphere — is such an easy place to lift off that it’s possible on that world for the whole lander to fly!
Dragonfly and the Mars Helicopter Scout, to scale. The rotor diameters are about the same size, but Titan’s thick atmosphere makes it easy to fly a much more capable vehicle.
The MSL Curiosity rover (which arrived at Mars in 2012) was lowered to the ground by a short-lived rocket “skycrane” (as Mars 2020 will be). One can think of Dragonfly as a lander with its own reusable rotor skycrane. However, while MSL’s rockets burned for only a tense 30 seconds before touchdown and it has since driven 18 km in six years, Dragonfly could fly farther than this in a single flight in less than an hour.
Titan is far from the Sun — 10 times farther than the Earth-Sun distance — and, taking Titan’s hazy atmosphere into account, there is 1,000 times less solar power reaching the surface. This makes solar power impractical, so a radioisotope power source must be used (as was the case for Cassini at Saturn).
The power source we propose is the MMRTG (Multi-Mission Radioisotope Thermoelectric Generator) — the same unit used on the MSL Curiosity rover and set for Mars 2020.
After several years in space, this unit would put out less than 100 watts of electricity. Importantly, it would also produce about two kilowatts of heat, which would help warm Dragonfly’s interior against the dense deeply cold Titan atmosphere, which is at 94K (-179°C). This heat is transferred the same way heat is extracted from a car engine, using a pumped fluid loop. These pumps have been used in space for particularly demanding thermal challenges, such as on Curiosity and the Parker solar probe.
During the Titan night — when Dragonfly is on the far side of Titan and can’t communicate with Earth — it would operate in a low power mode, mostly just “listening” with its seismometer and recording periodic weather readings.
This low power operation would allow Dragonfly to recharge its battery in time for sunrise.
Dragonfly would communicate with Earth directly (no help from a relay satellite) using a circular antenna deployed on its top deck; panorama cameras would also be mounted on the antenna, so there’s no need for a separate pointing mechanism. The antenna is not quite a dish; it’s actually flat, using an array of slots to generate a narrow beam much like the antenna used on Japan’s Akatsuki mission to Venus. This allows it to pack flat for streamlined atmospheric flight.
APL has a storied history of building spacecraft for challenging environments, but we are not planning this mission alone. In addition to partners providing the various scientific instruments (see Table 1), key expertise in rotorcraft design has come from Penn State University’s Vertical Lift Center of Excellence; in entry and descent systems (heat shield and parachutes) from NASA’s Langley and Ames centers; and in space navigation from the Jet Propulsion Laboratory.
Instrument | Lead | Technique |
---|---|---|
DrACO (Drill for the Acquisition of Complex Organics) | Kris Zacny, Honeybee Robotics | Dual rotary-percussive drills with custom bit. Pneumatic sample transfer system for rapid, cold transfer of drill cuttings to DraMS sample carousel. |
DraMS (Dragonfly Mass Spectrometer) | Melissa Trainer, NASA Goddard Space Flight Center | Mass spectrometer with laser desorption and thermal/gas chromatography front ends to analyze molecular composition of ices and organic compounds in sampled material. |
DraGNS (Dragonfly Gamma-Ray and Neutron Spectrometer) | David Laurence, Johns Hopkins APL | Pulsed neutron generator, with gamma-ray and neutron spectrometers to quickly measure elemental composition (carbon, nitrogen, hydrogen, oxygen, salts, etc.) of surface under lander. |
DragonCam (Dragonfly Camera Suite) | Mike Ravine, Malin Space Science Systems | Forward- and down-looking wide-angle cameras; pointable cameras for panoramas; close-up imagers to view drill sites and surface material. LED illuminators for color information and organic fluorescence detection. |
DraGMet (Dragonfly Geophysics and Meteorology Package) | Ralph Lorenz, Johns Hopkins APL | Wind, pressure, and temperature measurement and sensors for methane humidity and hydrogen gas. Electric field and physical properties of surface. Seismometer |
Table 1.
Flying on Titan is just like flying on Earth, but easier. Because Titan’s gravity is 1/7th of Earth’s — a little less than that of our own Moon (which is 1/6th of Earth’s) — the rotors don’t need to develop nearly as much thrust. Dragonfly on Titan weighs less on Titan than a typical person does on Earth, and because Titan’s atmosphere is four times denser than ours, the rotors can provide that thrust more easily (just as it’s easier to swim than to fly). All in all, if you took a terrestrial drone to Titan, it could hover using less than 3% of the power it needs on Earth.
Like most drones, Dragonfly has to be packaged in a heat shield. The heat shield has a cruise stage ‘backpack’ to provide communications and propulsion for course corrections on the way to Titan.
Titan’s atmosphere is mostly nitrogen like ours, but with a few percent of methane (and without any oxygen). Coupled with the low temperature, this makes the atmosphere a little less viscous, and means sound travels a bit more slowly. These factors and the density determine the best rotor shape and speed (for example, rotors become inefficient if their tip speed approaches Mach 1 — the local speed of sound).
In fact, the best blades for Titan are shaped rather like those for wind turbines on Earth. The chosen wing section doesn’t rely on particular surface smoothness, but is tolerant of dings or dirt. The low temperatures on Titan mean that the rotor motors must be warmed up before flight to soften the lubricants, just like the actuators on Curiosity.
Since Titan is a billion miles away, light (and radio signals) take more than an hour to get there. So, there is no hope of directly “joysticking” a flight from the ground. Thus, the flight control and the local navigation must be autonomous.
The basic autopilot would use an inertial measurement unit (IMU) with super-precise laser gyros and sensitive accelerometers. This is accurate enough for short flights, but for longer flights, cameras would be used to measure the flight speed over the ground. For even higher accuracy, Dragonfly’s cameras would match known landmarks. (There is no GPS on Titan, and Titan does not have a magnetic field, so compasses can’t be used).
Dragonfly’s autopilot uses sensors to follow a preassigned path.
This type of optical “terrain relative navigation” (TRN) has been recently developed for Mars and Moon landers, as well as terrestrial applications (APL has worked on digital scene matching for cruise missile guidance for decades). Even though Titan is 10 times farther from the Sun and has a hazy atmosphere, there is still enough light to take good pictures (same as the images from the Huygens probe attest; visibility was in excess of 10 km).
A ‘flash LiDAR’ measures the roughness of the terrain immediately under the lander, and so Dragonfly could find a safe spot to touch down by itself within a target zone specified by ground controllers in advance. Typically (except for the very first landing), Dragonfly would only be sent to a “safe” site it has already scouted from the air.
Dragonfly uses radar and LiDAR to sense the ground and find a safe landing spot. In the Namib dunefield shown, the flat interdune areas are 2-3 km across.
In fact, for the scientific traverse of multiple sites of different geological types, the plan is to ‘leapfrog’ to ensure optimum terrain for landing.
“Leapfrog” reconnaissance strategy lets landing sites be assessed before committing to them.
Departing site “A,” the vehicle would scout region “B,” and return to A which is known to be safe. Then, it will take off from A, scout region “C,” and return to land at B. This two-steps-forward-one-step-back strategy avoids the risk of heading into the unknown.
The first landing after Dragonfly enters Titan’s atmosphere and flies out from beneath its parachute-suspended heat shield would take place over a sand sea: a desert full of giant dunes. Images and radar data from Cassini have given us detailed maps of many areas. Although giant dunes sound forbidding, we know from sand seas on Earth (like the Namib or Arabian deserts which have dunes of exactly the same size and shape as Titan’s, up to 150 meters high) that the interdune areas — 2-3 kilometers wide — are typically very flat, often gravelly plains. So, even if Dragonfly emerges from its heat shield over a dune, it would need to fly only a couple of kilometers to find a flat spot to land.
Dragonfly releasing from its backshell (the parachute is above the top of the frame).
Once safely down on the ground, Dragonfly would point its antenna to Earth and begin transmitting data and making its first measurements for a few days before the Sun and the Earth set. Titan’s day (or “Tsol”) is 16 Earth days long, so the Titan night (when the lander is “sleeping”) is a week long, giving scientists time to examine the first data and refine plans for the next Tsol. Unlike the Mars rovers — where operators suffer “jetlag” by having to work on Mars time — Dragonfly’s operations can be comfortably planned (for the most part) during regular office hours!
The Dragonfly team is led by APL’s Dr. Elizabeth Turtle, who also leads the development of a powerful and agile camera on NASA’s Europa Clipper mission to Jupiter’s moon, and was responsible for planning Cassini’s camera observations of Titan. The team comprises scientists from across the US, as well as from several other countries, bringing expertise in Titan chemistry, geology, meteorology, and in various instrument techniques.
Although Dragonfly would provide exciting findings in a whole range of scientific areas — to say nothing of spectacular pictures — the main motivation of the mission is to understand Titan’s chemistry. We know there are abundant carbon-rich materials there, and there are also places where water has interacted with this material. When these kinds of reactions have been done in the laboratory in a few hours, many of the building blocks of life (such as amino acids which make up proteins in living things or pyrimidines — a molecule that stores information in DNA) can be made.
However, we don’t know, over thousands or millions of years, how far along the chain of chemical complexity these reactions can go toward the functions like information storage or energy generation in a planetary environment like Titan. We think these chemical processes happened on Earth once, but evidence of the details is lost. Titan is like a giant prebiotic chemistry laboratory where these crucial steps are being kept in deep freeze, waiting for us to pick them up.
Although Dragonfly doesn’t have a robotic arm (that on Curiosity is complex and heavy), it can interact with its environment in several ways. Its drills can acquire samples (using rotary-only or rotary-percussive action); the texture of the cuttings can be inspected with the cameras before deciding to ingest a sample. The percussion (“hammer”) function on a drill could also be operated to transmit vibrations which can be picked up by the seismic sensor to deduce the stiffness of the ground (the sound of drilling would also be measured by an onboard microphone).
A rotor can be spun up to various speeds to impose different levels of wind stress onto the ground, and by observing with the cameras and electric field sensors, the wind needed to make sand move can be measured. This “threshold wind speed” is an important parameter for decoding what the giant sand dunes mapped by Cassini tell us about Titan’s climate history.
Dragonfly’s drill systems (one on each of the two skids) are being developed by Honeybee Robotics of Pasadena, CA. The robust drill itself is evolved from that used by the Apollo astronauts on the Moon, with a specially designed drill bit for sampling Titan’s (softer) materials. The drill cuttings are transferred to the chemical analysis instrument by suction, much like a vacuum cleaner.
The instrument — a mass spectrometer to measure the size of molecules either zapped off a sample with a laser or cooked in a tiny oven — is quite similar to the corresponding instrument on the Curiosity rover which recently detected organic compounds on Mars.
Scientists would help decide whether to take a sample at a given landing site using information from another instrument: a gamma-ray spectrometer, which “illuminates” the ground with pulses of neutrons from a generator (a bit like an X-ray tube; again, the Curiosity rover has a similar neutron source) and measures the neutrons and gamma rays that are emitted. This radiation would tell us whether we are on a deposit of frozen water, and whether that ice is salty or not, or whether we are instead on organic-rich material.
One helpful feature of the Titan environment is that the special high-purity Germanium gamma-ray detector needs to operate below about 100K. Normally on space missions, such detectors need mechanical cryocoolers (essentially little refrigerators) to get this cold, but on Titan we can just hang the detector out in the cold breeze!
Of course, like any lander or rover, Dragonfly would carry a suite of cameras. Down-looking wide-angle cameras on the vehicle’s belly are used for mapping during flights, as well as for viewing the area under the lander to decide on sampling. Close-up imagers would be able to see individual sand grains and look at the sites where each drill will touch the ground. Although Titan’s haze bathes the dayside in dim red light, Dragonfly could take color close-ups using a multicolor “flashlight” with light-emitting diodes (LEDs) at night.
Vehicle | Dragonfly | Mars Helicopter Scout | Phantom 4 Drone | EHang 184 Aerial Taxi |
---|---|---|---|---|
Designer | Johns Hopkins APL** Laurel, MD | JPL Pasadena, CA | DJI Shenzhen, China | EHang Beijing, China |
World | Titan | Mars | Earth | Earth |
Gravity (m/s2) | 1.35 | 3.7 | 9.8 |
9.8 |
Air Density (kg/m3) | 5.4 | 0.02 | 1.2 | 1.2 |
Vehicle Mass (kg) | >400* | 1.8 | 1.4 | 360 (incl. 100 kg passenger) |
Number and Configuration of Rotors | 8 – Dual Quad | 2 – Coaxial | 4 - Quad | 8 – Dual Quad |
Rotor Diameter (m) | >~1* | 1.2 | 0.24 | 1.4 |
Flight Time (minutes) | Tens of minutes* | 2-3 minutes | 28 minutes | 23 minutes |
Recharge Time | 8 days | 1 day | 70 minutes | 1 hour |
Flight Speed (m/s) | ~10* (max range speed) | a few* | ~20 (max speed) | ~40 (max speed) |
Range | Tens of km* | 600m | (line of sight per FAA) | ~16 km |
Power Source | Battery/MMRTG | Battery/Solar | Battery/Mains | Battery/Mains |
Guidance Sensors | Radar, LiDAR, Cameras | Camera | GPS/Camera |
GPS |
Data Transmission Frequency | 8 GHz (X-band) direct to Earth | 900 MHz Zigbee to Mars 2020 rover | 2.4 GHz | |
Mission Duration | > 2 years | < 30 days |
**The APL-led Dragonfly team includes Penn State University, NASA, Honeybee Robotics, Malin Space Science Systems, and many other institutions.
*Some precise performance values have not been released or are undergoing refinement. Representative values are given here; data from Wikipedia, Dragonfly, DJI, and EHang websites.
Table 2.
One interesting feature of this is that Dragonfly would use ultraviolet (UV) LEDs because many organic compounds that we expect on Titan fluoresce; glowing blue or green when illuminated with UV. You can try this for yourself with a black light and tonic water (which contains quinine) or laundry detergent (the “brightening agent” is another fluorescent organic material). The design also includes forward-looking cameras for scouting from the air, and cameras mounted at the top of the communications antenna to make panoramic views of each landing site.
Finally, the instrument that I lead is a meteorology and geophysics package that would basically monitor a large suite of simple sensors like many SERVO readers are used to working with, such as temperature and pressure. We’d also measure the electrical capacitance under the vehicle skids which gives us a measure of the dielectric constant of the surface, telling us something about its texture and composition.
We measure wind speed by “hot film anemometry;” basically, heating a small cylinder and measuring how much “wind chill” the cylinder feels in different directions. Because the lander structure can distort the airflow slightly, we’ll actually have four wind sensors (one on each rotor hub), so that at least two are “upwind” of the vehicle and see undisturbed airflow. The wind sensors are also used to check that conditions are safe for takeoff.
Of course, we know much less about Titan than we do about Mars. This is, after all, one of the principal motivations for going there to explore! Titan has been mapped in some detail over more than 13 years by the NASA-ESA Cassini orbiter, which ended its mission in 2017. Furthermore, Cassini delivered the European Space Agency’s Huygens probe to Titan in 2005. Huygens parachuted down through Titan’s atmosphere for two-and-a-half hours to a comfortably soft landing, after which it operated for several hours before its batteries expired. Huygens imaged a small area of Titan at very high resolution and measured in situ the atmosphere composition and wind profile.
Cassini Radar image, about 200 km top-to-bottom, showing the long parallel ridges of dunes, 3 km apart, just like those in the Namib or Arabian deserts. The bright patches are low hills, that block the dunes or cause them to sweep around.
In fact, Dragonfly would reach Titan almost exactly one Titan year (29.5 Earth years) after Huygens and at a similar latitude, so the Huygens measurements are directly applicable. The winds near Titan’s surface as measured by Huygens are rather weak; typically, less than 0.5 meters per second (one mile per hour). Numerical climate simulations like the models used to predict our weather (but adapted for Titan) show that these conditions are typical.
Also, while Titan has a hydrological cycle of clouds and rain and lakes (with methane [liquefied natural gas] as the working fluid, not water), Cassini observations and the models show that rainstorms do not happen over the equatorial dunefields at this season. Even so, Dragonfly is designed to tolerate all conditions.
In fact, I first proposed the idea of a relocatable rotorcraft lander for Titan using a battery recharged by a radioisotope power source in 1999. (Actually, I was doing a study on airships, and while balloons and airships would work reasonably well on Titan, it became quickly obvious that it was actually heavier-than-air vehicles that could best exploit the Titan environment.) At the time, however, rotorcraft meant helicopters with all the mechanical complexity of their variable-pitch rotors, and the electronics and systems for autonomous flight were still in early development. Even the power source was notional. It was an idea before its time.
Fast-forward a couple of decades, and the key technologies are now in place. The MMRTG has flown and has well-established performance; the autopilots and machine vision and hazard avoidance systems have been developed for consumer drones, as well as NASA and other lunar and Martian lander programs; and the battery and motor technology has improved. Plus, scientifically, with 13 years of Cassini data, we now know Titan’s environment well enough to plan a mission that would take the next step to explore the prebiotic chemistry and habitability of this ocean world.
All the pieces have come together. I was privileged to have worked on Huygens — the spacecraft that made the first landing on Titan. With luck, I’ll also get to build Dragonfly, to make the second soft-landing, and the third, and the fourth, and ... SV
Ralph Lorenz is a scientist at the Johns Hopkins Applied Physics Laboratory and author of the books “Titan Unveiled” and the Haynes “Cassini-Huygens Owners’ Workshop Manual.” He can be reached at ralph.lorenz@jhuapl.edu.
Article Comments